
LII: Laser Induced Incandescence
Instrument for soot characterization
The LII method involves rapidly heating soot residing or passing within a laser beam path using a high energy (~100 mJ) pulsed laser source with duration typically less than 20ns. Elemental carbon particles (soot) interacts with the laser beam absorbing light which is converted into heat and increases the particle temperature. Particles are heated from the local ambient temperature to a temperature just below the soot vaporization or more accurately, sublimation temperature (approximately 4000 to 4400oK). Thermal radiation or incandescence emitted from the soot particles is measured using collection optics and photodetectors. With appropriate calibration and analysis of the incandescence signal, information on the soot volume fraction, primary soot particle size, and specific surface area may be obtained. Laser energy absorption by the soot particles and the subsequent cooling processes involve complex analysis of nanoscale light absorption, heating and mass transfer in time and space. The method is largely nonintrusive and is capable of making in situ measurements over a very large range of soot concentrations in flames and under ambient conditions using sampling.
In the Artium LII systems, a pulsed Nd:YAG laser producing light at 1064 nm is used to heat the soot particles. The laser wavelength is easily blocked from contributing to the incandescence signal that is generally observed in the spectral range of 400 to 800 nm using laser line filters. A critical requirement is to produce a laser beam intensity profile that is nearly uniform or top-hat. This ensures that all of the soot within the sample volume is heated nearly uniformly. The uniform beam intensity profile is produced by relay imaging the face of the laser rod to the measurement volume. Another approach is to use an aperture which clips the wings of the laser beam intensity and allows only the nearly uniform central part of the beam to pass. This aperture is relay imaged to the sample volume. Incandescence detection requires careful analysis and design of the optical system to achieve high collection efficiency, accurate representation of the sample volume, and transmission of the incandescence to the photodetectors. Incandescence signals typically increase to maximum intensity in a matter of a few nanoseconds and then decay over a period of time on the order of a microsecond. Thus, the photodetectors and high resolution analog-to-digital converters (ADCs) 5 must be able to respond to these short duration signals and accurately represent the amplitude and decay rate, if reliable measurements are to be realized. The Artium LII system has been carefully designed to achieve high resolution and high accuracy recordings of each of the LII signals.
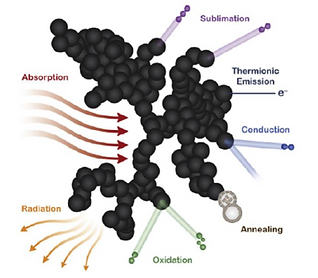
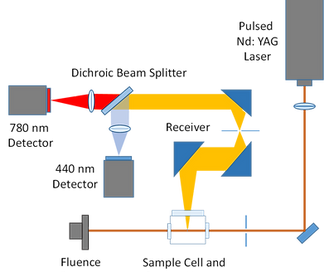

Heading 1
Laser Induced Incandescence Theory of Operation
Analysis of the incandescence signature requires a theoretical framework or model. Specifically, the laser heating process is assumed to be independent of particle size, and the emitted incandescence is nominally volumetric, when the following assumptions apply:
-
soot primary particles are small compared to the laser wavelength (Rayleigh regime)
-
laser heating increases the temperature of all particles at the same rate, regardless of size
-
when the particles reach the vaporization temperature, additional absorbed energy goes into vaporization rather than sensible energy, so that the particles remain at the same temperature for the duration of the laser heating period
-
vaporization causes negligible particle-size reduction, so that the incandescent radiation from the particles is independent of laser fluence above the vaporization threshold
Obtaining quantitative soot concentration measurements with the early or conventional LII methods required calibration using a soot source typically consisting of a laminar diffusion flame. The soot source which may have been measured using light extinction or other suitable “standard” to provide a reliable measurement of the soot concentration, was used in the calibration procedure. Unfortunately, the procedure was somewhat challenging, especially in determining what the actual soot concentration was when used as the calibration source. Furthermore, soot generated using different combustion means will have significantly different characteristics in terms of aggregate size, primary particle size, condensed soluble organic fraction (SOF), graphitization, etc.. For example, diffusion flames produce soot that may be expected to have different morphology and chemical characteristics than gas turbine, diesel, or other combustion generated soot. Added to that complexity was the requirement that the fluence be sufficiently high to raise the soot temperature to close to the sublimation temperature. Recall that soot incandescence depends on the mass concentration of soot and its temperature. Without a means for measuring temperature, the conventional LII methods needed to use the high fluence approach to minimize the soot temperature dependence on the incandescence signals. As a consequence, they generated a certain soot mass loss due to sublimation.
Because of the recent requirement to calibrate PM mass measurement devices using the NIOSH 5040 method, the need to produce repeatable soot characteristics and characterize the soot volume fraction using the Sunset OC/EC method has reappeared. The assumption is that the NIOSH 5040 method is highly reliable, precise, and accurate may be challenged. Moreover, CAST 13 burner soot or diffusion flame soot is known to have significantly different characteristics (volatile content, morphology, aggregate and primary particle size, graphitic characteristics, etc.). Thus, the response of the different PM mass measurement methods and especially LII need to be evaluated under these conditions. One primary challenge is to generate soot with characteristics similar to gas turbine soot. A further challenge is to ascertain what the relevant characteristics might be and then reproduce them in a concise and repeatable manner.
A professed advantage of Artium’s AC LII approach is that absolute intensity measurements are made and related to the soot volume fraction, primary particle size, and specific surface area. Reliably relating the incandescent signal to detector voltages that can be measured requires calibration to characterize the optical transfer function. This characterization includes collection efficiency of the receiver system, components in the optical path including beam attenuation systems, optical filters, and the response of the photodetectors (photomultiplier tubes) along with the electronics amplification components. We use an integrating sphere and calibrated photo-spectrometer as our optical calibration source. Integrating spheres consist of a light source and a sphere with its interior coated so as to produce uniform radiance. This method is described in detail in a later section on calibration.
Artium LII systems have shown good agreement between gravimetric measurements of soot, comparisons to other methods and to the NIOSH 5040 method, albeit with a correction factor to the slope of the response.